A pharmaceutical microcrystalline cellulose pellet is a small spherical agglomerate made of 100 % microcrystalline cellulose as a chemically inert material. Pellets are used as an excipient for tablet manufacture. Narrow particle size distributions are achieved with current production technologies. From a galenic point of view, an ideally defined and smooth surface speaks for the use of pellets in pharmaceutical formulations. In addition, pellets as a carrier system for active ingredients show particle size-dependent properties, such as fast and reproducible gastric passage, as well as uniform distribution in the respective section of the gastrointestinal tract during passage. Pellets are used in pharmaceutical formulations targeting oral dosage formas, such as capsules, MUPS (Multiple Unit Pellet System), or tablets.
Posts
Abstract
Pellets are one of multiparticulate pharmaceutical forms and can offer numerous technical and biopharmaceutical advantages compared with single dose unit formulations, e.g. tablets and capsules. This study aimed at formulation of controlled-release pellets of doxazosin mesylate (DM), a widely used treatment for antihypertensive and benign prostatic hyperplasia. DM was loaded onto microcrystalline cellulose CELLETS® pellets using hydroalcoholic solution and alcoholic suspension layering techniques to achieve a minimum drug load of 4 mg DM/g pellets. DM-layered CELLETS were coated by Aquacoat dispersion (ready-made ethylcellulose dispersion) using a coating pan technique as a simple and widely utilized technique in pharmaceutical industry. Controlled-release DM-layered pellets showed a release profile comparable to the controlled-release commercial product Cardura® XL Tablet. Also, the mechanism of DM release from Aquacoat CELLETS® was mathematically modeled and imaged by scanning electron microscopy to elucidate drug release mechanisms from the prepared pellet formulations. Accelerated stability studies of the prepared pellets were performed under stress conditions of 40 °C, and 75 % RH for 3 months. In conclusion, preparation of controlled-release DM-layered CELLETS® is feasible using a simple and conventional coating pan technology. Read more about controlled-release doxazosin mesylate pellets.
References
H. A. Hazzah, M. A. EL-Massik, O. Y. Abdallah & H. Abdelkader, Journal of Pharmaceutical Investigation (2013), 43:333–342. doi:10.1007/s40005-013-0077-0
Additional information
CELLETS® are perfect starter beads for coating and layering of API, such as doxazosin mesylate. Multilayer formulation attempts enable defined release profiles and improved bioavailability. Check different pellet sizes from 100 µm to 1400 µm which fit to your formulation.
Need formulation services?
Contact our partner Glatt Pharmaceutical Services!
This article “Amorphous Solid Dispersions Layered onto Pellets – An Alternative to Spray Drying?” is an excerpt from the publication of Neuwirth et al., Pharmaceutics 2023, 15(3), 764; https://doi.org/10.3390/pharmaceutics15030764.
Abstract
Materials
Pellet Properties | |
---|---|
d50 (xc min) [µm] | 1123.44 (±7.36) |
SPAN | 0.166 (±0.002) |
b/l | 0.893 (±0.000) |
SPHT | 0.956 (±0.001) |
Particle density [g/cm3] | 1.452 (±0.016) |
Sm [cm2/g] | 36.41 (±0.29) |
Table 1. Pellet properties of Cellets 1000. d50: mean particle diameter determined by the particle width; SPAN: width of the particle distribution; b/l: aspect ratio; SPHT: sphericity; Sm: specific surface area.
Conclusions
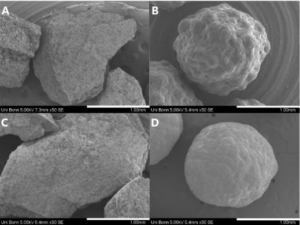
Figure: Amorphous solid dispersions.
[1] Neuwirth et al., Pharmaceutics 2023, 15(3), 764; https://doi.org/10.3390/pharmaceutics15030764
Abstract
This case study is a short abstract on spouted bed characteristics, following closely findings in the publication by J. Vanamu and A. Sahoo [1].
Spouted bed systems are of highest importance for all powder processing industries, and more specific in pharmaceutical industry for coating and drying in pellet technologies [2]. These systems offer manufacturing particularly fine and temperature-sensitive particles from small to large scale: laboratory systems are capable of processing product volumes of very few grams, while production systems can handle capacities of several tons [3].
But how to control conditions in spouted beds for efficient process applications, like mixing, coating, or drying?
There might be certain reasons, that the hydrodynamic behavior of the spouted bed in the pharmaceutical industries is less investigated. The referred publication shed some light on the hydrodynamic characteristics of a spouted bed where the MCC Spheres (CELLETS®) are adopted as the bed material. These starter cores are ideal model systems due to their perfect sphericity and zero-level friability. At the same time, smooth and defined surface structure initiate perfect modelling conditions in the spouted bed dynamics.
Material
CELLETS®, made of 100% Microcrystalline Cellulose, have been used as bed material. The physical properties of the CELLETS® are shown in Table 1. The CELLETS® particle morphology is represented in Figure 1.
Parameter | Value |
CELLETS® 700 and CELLETS® 1000 | |
Size distribution | 700-1000 µm (CELLETS® 700)
1000-1400 µm (CELLETS® 1000) |
Bulk density | 800 kg/m3 |
Particle sphericity | > 0.9 |
Void fraction | 0.42 |
Geldart classification | B |
Table 1: Physical properties of the CELLETS®.
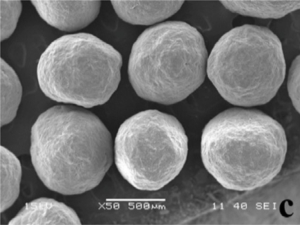
Figure 1: SEM micrographs of CELLETS® 700, found in [1].
Spouted bed: experiment setup
There are some international players on the market of spouted bed technologies, such as Glatt which seems to be the major one (Figure 2). In this framework, a self-made setup is used for experiments. The experiments that have been carried out in a column, which is fabricated from a Perspex sheet. This column consists of a cylindrical section of height 0.53 m and a diameter of the cylinder of 0.135 m. The column further converged the diameter of the cylinder to 0.05 m as a conical bottom having a length of 0.47 m. The spouting air is supplied by a compressed air line is controlled by a gas regulator. The airflow is controlled by a gate valve and a mesh plate having a mesh size less than the size of the bed material is employed as a separator preventing the backflow of the bed material. Images are captured using a high-speed video camera to gain more details of the hydrodynamic characteristics of the flow pattern inside the spouted bed geometry.
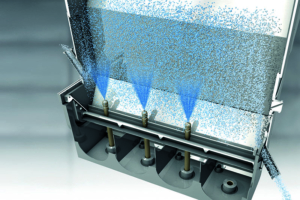
Figure 2: Scheme of a spouted bed (Glatt, Germany).
Experiments & spouted bed results
Experiments are carried out with three different static bed heights of shallow depth wherein the bed height is in the range of factor 2-3 of the Inlet diameter using two different particle distribution classes at 500-710 µm and 700-1000 µm, respectively. Analyzed parameters are the pressure drop across the bed, the bed expansion ratio, and the clusters concerning the superficial gas velocity are focused in the following.
J. Vanamu et al. found that the “bed expansion ratio increases with increasing superficial gas velocity until the onset of external spouting, further increase in the superficial gas velocity, the bed expansion ratio decreases. With increasing the volume of bed, the bed expansion ratio decreases. In a larger volume of bed, the particles tend to spout into the freeboard region rather than expanding with higher superficial gas velocity”. Initial spouting is symmetric, but with increasing superficial gas velocity spouting becomes asymmetric, and asymmetry is more pronounced or starts at lower superficial gas velocities for smaller particles. This agrees with existing theories of hydrodynamic behavior in a fluidized environment. Respecting the necessarity of a proper flow behavior for mixing, coating or drying applications in drug processing, symmetric spouting is essential. In turn, the superficial gas velocity may be kept low.
In case that high superficial gas velocity regimes are required for the operations a draft tube may be installed within the column to achieve the symmetric spout formation.
Summary
This case study highlights the Hydrodynamic behavior of MCC spheres in a spouted bed using image processing method. MCC spheres in the range between 500-710 µm and 700-1000 µm had been employed. All spheres showed a symmetric and asymmetric spouting in the spouted bed. With increasing superficial gas velocity, the fully suspended particles are limited to a certain height in the freeboard region due to the gas-solid crossflow. A change from symmetric to asymmetric spouting is observed with increasing superficial gas velocity.
Keeping the conditions efficient for the mixing, coating or drying applications requires finally to suppress high superficial gas velocities, or changing the setup in such way, that symmetric spouting conditions are kept upright even at higher superficial gas velocities.
References
[1] J. Vanamu and A. Sahoo, Particuology 76 (2023) 101
[2] L. A. P. de Freitas, Particuology 42 (2019) 126
[3] Glatt GmbH, Binzen, Germany. Online on Nov 8, 2022: Spouted bed systems – Glatt – Integrated Process Solutions
Great thanks to Arihant Innochem Pvt. Ltd. who supplied and donated CELLETS® for this study.
Abstract
Multiparticulates made of pellets are ideal dosage forms to be used in pediatrics. Having the suitability of paediatric consumers in mind, formulations of small-sized pellets offer a valuable base for increased compliance and improved age-appropriately dosage form. Due to their round shape of pellets, smooth surface area and narrow particle size distribution they can easily be functionally coated [1] to achieve e. g. a taste masking, enteric protection or the controlled release of the active pharmaceutical ingredient (API) in defined parts of the gastro-intestinal (GI) tract. The release profile then often depends on the coating weight gain (thickness) and composition of the functional coating.
Coating weight gain, manufacture and analysis of pellets
A well soluble drug was used as model API. In a first approach, pellets were produced applying the ProCell technology, a direct pelletization process allowing the production of highly drug loaded matrix pellets (here 95%) in a spouted bed. Two types of pellets were produced: A) with a poly amino saccharide-based binder, followed by a cellulose based seal coating and B) with a polyacrylic acid-based binder, followed by a pH-depending coating. In a second approach the API was layered onto inert starter cores (MCC, CELLETS® 200) by the aid of a cellulose based binder and antitacking agent applying the Wurster technology targeting a drug load of 50 %, followed by a pH-depending coating (C). All three pellets-based populations were functionally coated by a pH-independent sustained release polymer. Samples were taken at pre-defined coating levels for dissolution testing. For API layering and coating a GPCG 1.1 with a 6” Wurster insert was used. Direct pelletization was performed in a ProCell 5. Particle size distribution (PSD) analysis was performed by Eyecon2TM. The particle size is given as numeric or volumetric distribution (e.g. Dn50 or Dv50). The specific surface area is calculated by measuring the true density by gas pycnometry and the Sauter diameter by Laser diffraction. Dissolution was measured in the acid stage (0.1 M HCl), in buffer pH 5.5 and in buffer pH 7.2 over 300 min. The API should not be released in the first 180 min. Between 210 min and 240 min an increased drug release is expected. The dissolution rates at 225 min were compared for the coating levels at 10, 15 and 20 %.
Results
With increasing coating weight gains decreasing dissolution rates at 225 min were measured for the sustained release coating with a good linearity. Matrix PEL (A) show higher dissolution rates comparing the same coating levels than Matrix PEL (B), Wurster pellets showed the strongest decrease with increasing CWG, table 1, figure 1. This correlation was not observed for pH-depending coating (data not shown).
Dv 50 [µm] | Dn 50 [µm] | PSD mean [µm] | Specific surface area [m2/g] | ||
A | Matrix PEL | 496 | 475 | 481 | 0,00980 |
B | Matrix PEL | 461 | 427 | 425 | 0,01210 |
C | Wurster PEL | 414 | 396 | 401 | 0,01100 |
Table 1. PSD data and specific surface area of starter beads before functional coating.
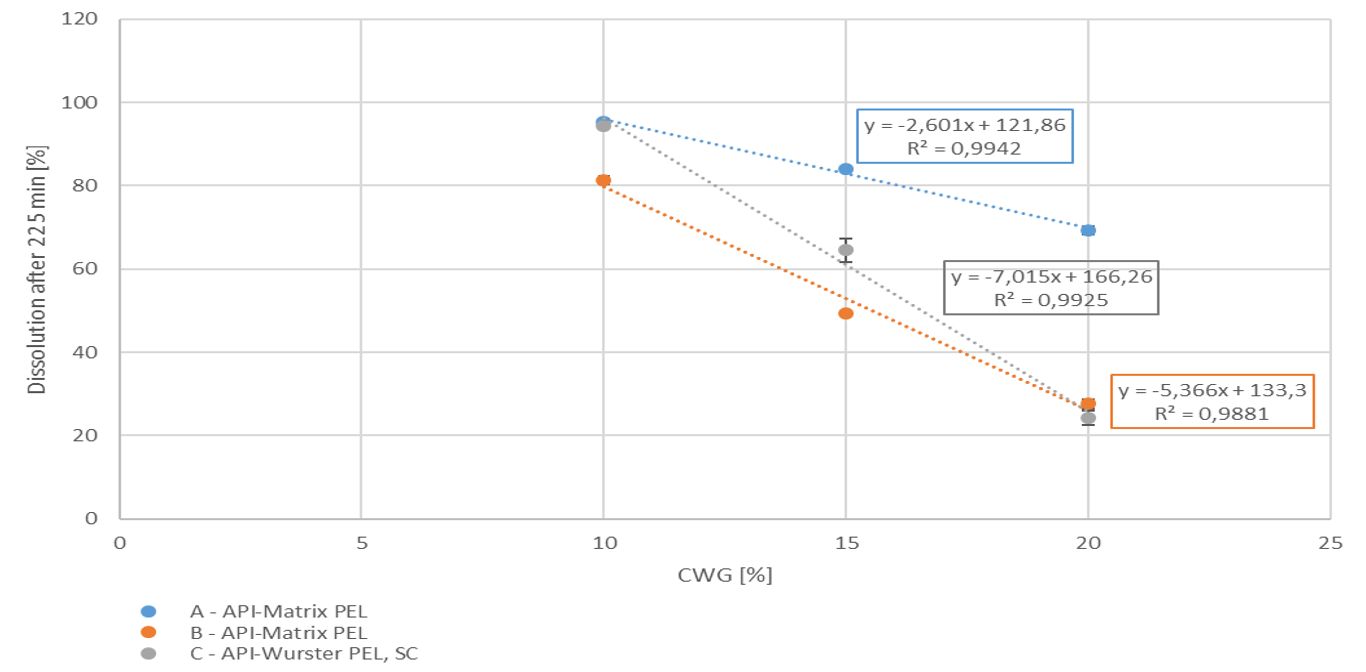
Figure 1. Dissolution at 225 min vs. coating weight gain (CWG)
Summary
Drug loaded pellets were prepared either as matrix pellets applying the ProCell technology, or by layering of starter cores applying the Wurster technology. Both populations were coated with different coating levels of a sustained release functional coating, resulting in decreasing dissolution rates with increasing coating weight gain. Due to the good correlation between coating weight gain and dissolution profile a prediction of the dissolution rate might be possible for pre-defined coating levels. These findings are a crucial step towards novel paediatric formulations with improved dissolution profiles and dosage safety.
References
[1] Palugan, L.; Cerea, M.; Zema, L.; Gazzaniga, A.; Maroni, A. Coated pellets for oral colon delivery, Journal of Drug Delivery Science and Technology 25, 1 – 15 (2015).
This study was presented on 14th annual EuPFI conference, Rome, Italy.
Abstract
This case study on Atomoxetine HCl pellets is a short abstract of the publication by Y.D. Priya et al. [1].
Atomoxetine is a medication used to treat attention deficit hyperactivity disorder (ADHD) [2]. The API is marketed under the trade names Atomoxetine, Atomoxe, Agakalin, and Strattera (initially launched) [3]. Atomoxetine is an extremely bitter API. As being initially launched for children as capsules or tablets, the paediatric compliance by improved taste-masking and the simplified administration to paediatrics are in focus of this study.
A multi-unit particulate pellet coating (MUPS) was selected as oral dosage form. The fluidized bed technology (with Wurster column) was employed for coating and layering processes. This is a well-known technology, which Is for instance offered by Glatt. Starter cores were coated with the API, followed by layering with a polymeric coating for which realized the taste-masking.
Atomoxetine layering
Starter cores are made of Microcrystalline Cellulose (MCC) in sizes comparable to CELLETS® 200, while a fair efficiency of drug layering was observed with the combination of HPMC (Hydroxypropyl methyl cellulose) and HPC (Hydroxypropyl cellulose) as binders. The composition of API layering is presented in Table 1. The drug dispersion was sprayed onto the MCC pellets with an inlet temperature between 50 °C and 55 °C and a fluidized bed temperature between 35 °C and 40 °C.
API layering material | Composition |
Starter core | |
MCC pellets | 58.00 |
API layering | |
Atomoxetine HCl | 25.00 |
Hydroxypropyl methylcellulose | 3.50 |
Hydroxypropyl Cellulose | 3.50 |
Low-Substituted Hydroxypropyl Cellulose | 5.00 |
Talc | 5.00 |
Purified Water | Qs |
Total weight (mg) | 100.00 |
Table 1: Formulation of API layered pellets.
Taste-masking coating
The polymeric taste-masking layer is made of a methacrylate co-polymer (Eudragit EPO) providing an excellent coating with taste masking properties for fine particles and tablets. The composition of the taste-masking suspension is shown in Table 2. The inlet temperature is between 40 °C and 45 °C, and fluidized bed temperature is between 25 °C and 30 °C.
Polymeric coating material | Composition |
Drug Layered pellets | 100.00 |
Eudragit EPO | 25.00 |
Sodium Lauryl Sulfate | 2.500 |
Stearic acid | 3.750 |
Talc | 6.25 |
FD&C Yellow No. 6 | 0.50 |
FD&C Red No. 3 | 0.05 |
Purified Water | Qs |
Total weight (mg) | 138.050 |
Table 2: Formulation of polymeric coating suspension.
The efficiency of taste-masking was benchmarked by a bitterness rating on human volunteers. Figure 1 shows, that the taste sensitivity identifies a bitterness at 6 µg/ml API concentration and an extreme bitterness at 7 µg/ml API and higher concentration. Thus, the threshold bitterness of Atomoxetine HCl is 6 µg/ml.

Figure 1: Concentration of drug solution (µg/ ml). Bitter intensity ratings from no bitterness (green), bitterness (blue), extremely bitter (red).
All the volunteers felt bitter taste when the drug layered pellets were coated with 6.25 mg of Eudragit EPO. Whereas in the pellets coated with 12.5 mg and 18.75 mg of Eudragit EPO, bitter taste was masked up to 15 seconds after keeping the tablet in the mouth, and later all the human volunteers felt bitter taste. When the concentration of Eudragit EPO was increased to 25 mg, the bitter taste of Atomoxetine HCl was completely taste-masked and no volunteer was felt bitter taste.

Figure 2: In-Vivo Taste evaluation in healthy human volunteers.
Figure 3 depicts the entire particle size of a taste-masked MCC pellet coated with the Atomoxetine drug layer and 25 mg of Eudragit EPO. The average particle size of the taste-masked pellets is between 180 µm and 250 µm, assuming, that no gritty feeling of particles in patient’s mouth will appear. It should be said, that a micronization of Atomoxetine HCl was deemed to be necessary for the drug layering process. Micronization minimized the surface roughness of the API layered pellet so that an efficient taste-masking coating can be applied.
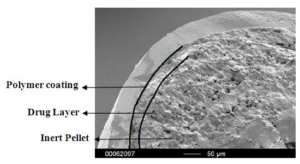
Figure 3: SEM picture of cross section of a Taste masked pellets coated with 25 mg Eudragit EPO.
Summary
MCC pellets in the size of about 200 µm were layered with Atomoxetine. HPMC and HPC were used as binders, realizing a precise surface definition for a subsequent taste-masking coating. The taste-masking was most efficient at a polymeric concentration of 25 mg. Keeping the size of the coated pellets below 300 µm avoids a gritty feeling and thus increase the patient’s compliance.
This study by Priya et al. indicated that the fluidized bed process produced the most appropriate taste masked pellets of Atomoxetine HCl for oral disintegrating tablets.
References
[1] Y.D. Priya et al., Int J Pharm Pharm Sci, (6) 7, (2014) 110-115
[2] “Atomoxetine Hydrochloride Monograph for Professionals”. Drugs.com. American Society of Health-System Pharmacists. Archived from the original on 4 April 2019. Retrieved 22 March 2019.
[3] ROTE LISTE 2017, Verlag Rote Liste Service GmbH, Frankfurt am Main, ISBN 978-3-946057-10-9, (2017) 162.
Abstract
Multidimensional Correlation of Surface Smoothness and Process Conditions is a necessary attempt to better understand, optimize and outperform process steps of drug formulations. Particle coating and layering in a fluidized bed process is a main attempt in pharmaceutical industry for drug production for modern oral dosage forms. The precise knowledge of control process parameters leads to high surface control of the drug-loaded particles and therefore is crucial for the quality and yield of production in a more general aspect. This application note presents a multidimensional attempt by Orth et al. [1] to correlate particle surface structure morphology and process conditions in a fluidized bed layering spray granulation. CELLETS® 500 are used as spherical, high-quality starter cores.
About fluidized bed process conditions
Fluidized bed processes are used in pharmaceutical, food and agro industries. Solid particles are transported in a defined gas stream inducing fluidized bed conditions. Solid-containing dispersions or liquids are sprayed onto the fluidized particles. Variable settings of process parameters allow particle layering, coating, coalescence and agglomeration. This point seems to make the fluidized bed becoming a universal process for particle processing, but also requests deeper knowledge about the desired process parameter settings: The goal is a stable, high-quality, high-output process.
Standard process parameters are:
- liquid spray rate (m1)
- fluidization air flow rate (Vair)
- fluidization air temperature (Tin)
- spray air temperature (Tat)
- spray atomization pressure (pat)
Beside the spraying process, also the drying process plays an important role. By drying, moisture, sticky conditions and flowability are strongly influenced. Hampel [2] analyzed in her doctoral thesis the importance of the drying process using CELLETS® 200 as model particles.
Technology, Materials and Analysis
The coating experiments were carried out in a ProCell® 5 LabSystem with the fluidized bed process chamber GF3 (Glatt GmbH, Germany) as shown in Figure 1. The ProCell® 5 LabSystem is designed for testing of spouted bed and fluidized bed processes in the single kg-scale.
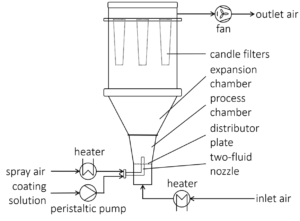
Fig.1: Sketch of the experimental fluidized bed setup (Procell® 5 LabSystem with GF3 chamber).
As Materials, pellets made of 100% microcrystalline cellulose (CELLETS® 500) are employed as perfect starter cores. These pellets provide smooth and defined surface properties, chemical inertness, robustness and a high degree of sphericity. Specific properties of CELLETS® 500 for this study are shown in Table 1. The roughness is at 1.5 µm and therefore delivers perfect initial conditions for controlled spray granulation.
Property | Value |
Sauter diameter | 639 µm |
Sphericity | 0.96 |
Surface roughness | 1.5 µm |
Solid density | 1.445 g/cm3 |
Table 1: Properties of CELLETS® 500.
As spray liquid, a 30 wt% sodium benzoate solution was injected into the process chamber. The mass ratio of spray liquid to starter cores was 1:2. Different coating conditions have statistically been driven. In turn, the spray-coated particles show different surface structures (Figure 2a-d).
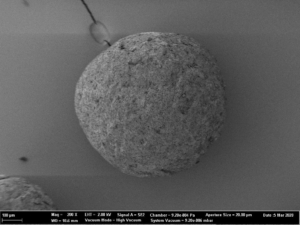
Fig.2a: SEM images of CELLETS® 500 particles coated with sodium benzoate at process conditions as printed in Table 2 (bold).
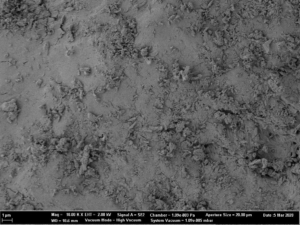
Fig. 2b: Close-up SEM images of CELLETS® 500 particles coated with sodium benzoate at process conditions as printed in Table 2 (bold).
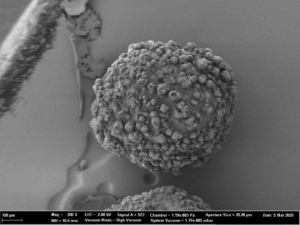
Fig. 2c: SEM images of CELLETS® 500 particles coated with sodium benzoate at process conditions as printed in Table 2 (italic).
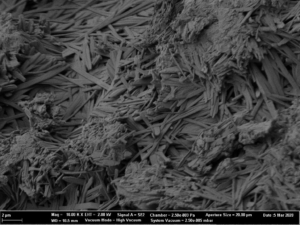
Fig. 2d: Close-up SEM images of CELLETS® 500 particles coated with sodium benzoate at process conditions as printed in Table 2 (italic).
Parameter | Controlled values |
liquid spray rate (m1) | 10 | 15 | 20 |
fluidization air flow rate (Vair) | 80 | 105 | 130 |
fluidization air temperature (Tin) | 50 | 85 | 120 |
spray air temperature (Tat) | 20 | 70 | 120 |
spray atomization pressure (pat) | 0.5 | 1.75 | 3.0 |
Table 2: Process parameters and values used in coating experiments.
The coated particles were analyzed regarding their surface roughness via laser scanning microscopy (VK-X160K, Keyence, Japan). Additional images were obtained with a scanning electron microscope (Supra VP55, Zeiss, Germany).
A 3D-profile of the particle surface was created and evaluated in a defined measurement area. Roughness analysis can be performed through several parameters as defined in DIN EN ISO 4287:2010-07 (2010) and DIN EN ISO 25178-2:2012 (2012). In this attempt, the arithmetical mean height was used as roughness quantifier. The roughness was correlated to the process parameters and the resulting linear correlation was rigorously analyzed using a principal component analysis.
Results
A linear regression model is fitted to the roughness data using the ordinary least squares method. This enables to create a linear model connecting the chosen process parameters to the surface roughness of the coated particles. It is mentionable, that in this attempt the fluidization air flow rate and the spray air temperature did not show a significant effect on the surface structure and were therefore removed from the model.
Process conditions: Influence of the liquid spray rate
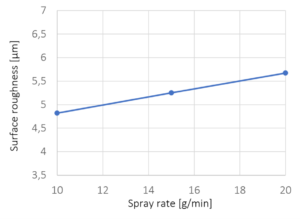
Figure 3: Surface roughness versus liquid spray rate. The crosses mark the experimentally investigated spray rates; line represents a linear interpolation.
The dependence of the surface roughness on the spray rate of the sodium benzoate solution is shown in Figure 3. A slight increase of surface roughness is identified for increasing spray rates. The main effect is considered to be influenced by the crystallization of sodium benzoate. Following, crystallization is higher at higher spray rates caused by lower evaporation due to higher liquid volumes in the process. The dependence of the crystallization of sodium benzoate on the drying conditions during fluidized bed coating was also observed by Rieck et al. [3] and Hoffmann et al. [4].
Process conditions: Influence of the fluidization air inlet temperature
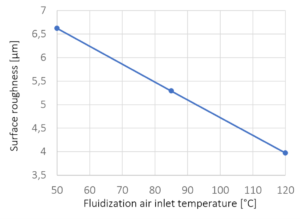
Figure 4: Surface roughness versus fluidization air inlet temperature. The crosses mark the experimentally investigated temperatures; line represents a linear interpolation.
An increase in the fluidization air inlet temperature results in a lower roughness of the coated particles and therefore in a smoother particle surface. The temperature of the fluidization air has a major impact on the drying conditions during the spray granulation process. As an increased temperature causes reduced relative humidity, the heated air can absorb a larger amount of water, which results in a high drying rate. Crystal growth of spray droplets is reduced by fast evaporation times and short drying times.
Process conditions: Influence of the atomization pressure
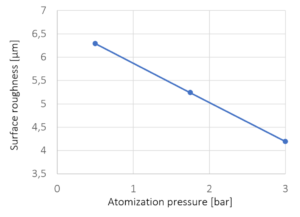
Figure 5: Surface roughness versus spray atomization pressure. The crosses mark the experimentally investigated pressures; line represents a linear interpolation.
With increasing atomization pressure from 0.5 bar to 3.0 bar, the surface roughness is decreasing. The pressure of the spray air strongly influences the droplet size and velocity. With increasing atomization pressure, the droplet size and size distribution decreases while the droplet velocity increases which in causes a more homogeneous spreading and promotes smoother surface coatings.
Summary
CELLETS® 500 are used as model particles for analyzing the surface roughness of coated particles dependent on process conditions in a bottom-spray process. As the results suggest, a high surface roughness is achieved at low fluidization air temperatures, low atomization pressures and high spray rates of the coating solution. Conversely, at high air temperatures, high spray pressures and low liquid spray rates, particles with smooth and compact surfaces are produced.
Acknowledgement
Prof. Stefan Heinrich and his team are gratefully acknowledged for serving content for this note:
Hamburg University of Technology
Institute of Solids Process Engineering and Particle Technology
Contact: Prof. Dr. Stefan Heinrich
Denickestrasse 15, 21073 Hamburg, Germany
Tel: +49 40 42878 3750
E-mail: stefan.heinrich@tuhh.de
Website: https://www.tuhh.de/spe/
The authors got funding from the German Research Foundation within the DFG Graduate School GRK 2462 “Processes in natural and technical Particle-Fluid-Systems (PintPFS)” (Project No. 390794421) and funding from BASF SE.
CELLETS® 500 were sponsored by HARKE Pharma.
References
[2] N.A. Hampel, Dissertation, Otto-von-Guericke-Universität Magdeburg, 2015. DOI:10.25673/4340
Abstract
Patients with dysphagia may have obstacles to swallow tablets or large multiparticulates. The former dosage form can even not be crushed in case that the tablet exhibits a modified release or taste-masking profile through outer layering. As a solution, so called jelly formulations may be a valuable attempt. Jellies are delivery vehicles incorporating sustained release microparticles for patients with dysphagia. This case study investigates a modified release formulation based on Gliclazide. Gliclazide is used to treat diabetes mellitus type 2. In combination with selected excipients, a jelly-like appearance is composed. Micropellets made of microcrystalline cellulose (Cellets®) are used as API carrier systems.
Goals and Formulation of a Gliclazide drug
The goal is to investigate a revolutionary method for geriatrics with dysphagia or potentially for paediatrics based on jelly-like formulations. The formulation should carry an API such as Gliclazide and show a modified release profile.
Free-standing jellies are formulated by mixing sodium alginate (0.5 % w/v with another polymer, and 1 % w/v w/o polymer), with an aqueous solution of dicalcium phosphate dihydrate (0.1-1 % w/v).
Soft granular jellies are formulated by preparing an aqueous sodium alginate (0.5-2 % w/v) solution with or without the presence of another polymer and by later adding an aqueous calcium chloride solution (0.1-0.3 % w/v).
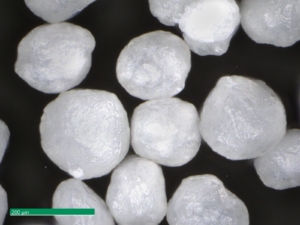
Figure 1: Image of MCC micropellets (Cellets® 100).
MCC micropellets (Cellets® 100, Figure 1) are used as drug carriers. Gliclazide is layered onto the starter beads using a Wurster fluidized bed coater (Mini-Glatt, Glatt GmbH, Germany), so that a 50 % drug loading weight gain was reached. The overall final drug load including the functional layer is 21 % w/w. The composition of the layering suspension is given in Table 1.
Material | QTY |
Starter pellet: Cellets® 100 | 100 g |
API: Gliclazide | 10 % w/w |
Aqueous vehicle for API: | |
Hypromellose | 1 % w/w |
Talc | 1.9 % w/w |
Coating of API layered pellets: | |
Water | |
Eudragit® NM 30 D | |
Talc | |
Functional coating: | |
Magnesium stearate | |
Silicon dioxide |
Table 1: Formulation for Gliclazide layered starter pellets: starter pellets, aqueous API layering, release profile coating, functional coating.
Although the formulation contains several coating and layering processes, the processed micropellets stay smooth in surface, show a high sphericity and narrow size distribution.
Size distribution and dissolution profiles of Gliclazide microparticles
Polymer coated micropellets with CL25 (coating level 25 %) are shown in Figure 2. The yield of polymer coating and the final D50 values of the micropellets are displayed in Table 2.
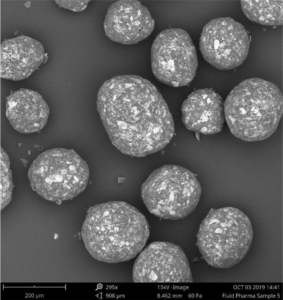
Figure 2: SEM image of layered Gliclazide sustained release micropellets with a weight gain at 25 % (CL25).
Depending on the polymer coating, micropellets show a different Gliclazide release profile as shown in Figure 3: With increasing weight gain, the dynamics of Gliclazide release are slowed down. A comparison to Diamicron SR tablets in a pH 7.4 phosphate buffer, the CL25 formulation results in an adequate release profile.
Micropellet | Size D50 [µm] | Yield [%] |
Starter pellet (Cellets® 100) | 160 ± 2.1 | |
Micropellet at CL16 | 173 ± 3.6 | 98.4 |
Micropellet at CL20 | 185 ± 2.4 | 99.3 |
Micropellet at CL25 | 198 ± 4.3 | 99.0 |
Micropellet at CL60 | 208 ± 6.7 | 98.7 |
Table 2: Particle size of the micropellets with and without layering. CL = coating level / weight gain in [%]. The yield for the polymer coatings at respective weight gains.
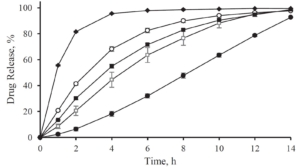
Figure 3: Gliclazide release from layered micropellets at coating levels 16 % (filled diamond), 20 % (open circles), 25 % (filled squares) and 60 % (filled circles) and the commercial Diamicron SR tablets (open squares) in phosphate buffer pH 7.4.
Incorporation of the Gliclazide microparticles into jellies
The incorporation of sustained release Gliclazide microparticles into the Jellies is realized through mixing the required quantity of microparticles with polymers (sodium alginate or polymer mixture).
Sodium alginate is known to form gels in the presence of calcium ions at room temperature. Depending on the formulation, granular jellies (soft and easy to flow) or free-standing jellies (“ready-to-eat”) are formed. Formulations of jellies with and without API layered micropellets are shown in Figure 4. Incorporating the micropellets into the jellies did not cause a visual change in color or appearance. The API was kept inside the jellies. Also physical-chemical properties such as the gel strength, the texture, and the oral transit time in an in-vitro swallowing simulator are remained unchanged.
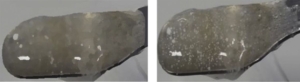
Figure 4: Images of a Jelly without (left) and with incorporation of sustained release micropellets (right).
Figure 4: Images of a Jelly without (left) and with incorporation of sustained release micropellets (right).
A release profile of Gliclazide with a coating level of 25 % in a jelly formation is shown in Figure 5. In comparison to a reference release profile of a Diamicron 30 mg SR tablet, the coated micropellets show a competitive behavior as already discussed in Figure 3. After incorporating into the jelly formation, the release profile is decaying. Obviously, the intact and also the fragmented jelly formulation show comparable dynamics. In order to obtain a comparable release profile than with the non-formulated micropellets, a coating level of down to 20 % is required.
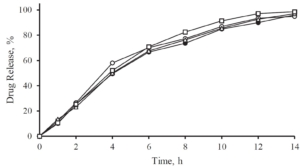
Figure 5: Gliclazide release from coated microparticles and in combination with Jellies in a pH 7.4 phosphate buffer. Diamicron 30 mg SR tablet (open triangle), no jelly at CL25 (closed triangle), jelly formulation (intact) incorporated with CL25 (closed circle), jelly formulation (fragmented) incorporated with CL25 (open circle), jelly formulation (intact) with CL20 (open square).
Summary
Sustained release Gliclazide micropellets with a final particle size D50 of less than 200 µm are successfully formulated with a 99 % production yield and adjustable drug release profiles.
The micropellets are based on Cellets® 100 and present an excellent surface smoothness, high sphericity and narrow size distribution. They were successfully incorporated in jelly formulations. This novel drug delivery platform is a suitable vehicle for the administration of sustained release microparticles. It is a valuable attempt to replace the commonly used thickened fluids for dysphagia patients.
Acknowledgement
Dr. Fang Liu and her team are gratefully acknowledged for serving content for this note.
Fluid Pharma Ltd
Contact: Dr. Fang LIU
College Lane, Hatfield, AL10 9AB, UK
Tel: +44 1707 28 4273
+44 796 3230 628
References
[1] S. Patel et al., Journal of Pharmaceutical 109 (2020) 2474-2484.
Abstract
Modified drug release formulations for suspensions are a perfect solution for children and patients with swallowing difficulties. In many cases, these formulations are based on pellets serving as starter beads. In this report, an attempt on microparticle coating by Mohylyuk et al. [1] is described. Herein, small scaled microcrystalline cellulose pellets (Cellets® 90 and Cellets® 100, Table 1) in the size range smaller than 150 µm are used. Through a modified Wurster fluidized bed process, a yield of 99 % was reached.
Starter materials | PSD (> 85 %) |
Cellets® 90 | 63-125 μm |
Cellets® 100 | 100-200 µm |
Table 1: Size distribution of Cellets® as starter beads in this formulation.
Goals and Formulation
The goal is to investigate a revolutionary platform for sustained-release microencapsulation using the industrial fluidized bed coating technology. Significant challenges of particle cohesion in the process shall be avoided by applying a small quantity of dry powder glidant periodically during the coating process. A highly water-soluble drug, which is metoprolol succinate, is reproducibly microencapsulated on pellet technologies with total pellet sizes of less than 200 µm and a drug release time of 20 hours.
Excipients for extended release profiles
For obtaining a sustained release profile, polymethacrylate-based copolymers, Eudragit RS/RL® 30 D and Eudragit® NM 30 D, were used in combination with a range of anti-tacking agents. The coating onto placebo Cellets® 100 starter beads was performed in a fluidized bed coater with a Wurster insert (Mini-Glatt, Glatt GmbH, Germany) in order to analyze the release profile. Process parameters are shown in Table 2. A small quantity of dry powder glidant was periodically added during processing, so that particle cohesion was eliminated. The optimized excipient composition for the desired release profile is achieved by testing 10 different compositions.
Parameter | Value |
Inlet air temperature | |
Eudragit RS/RL® 30 D | 35-40 °C |
Eudragit® NM 30 D | 30-35 °C |
Product temperature | |
Eudragit RS/RL® 30 D | 25-30 °C |
Eudragit® NM 30 D | 18-20 °C |
air flow rate | 18 m3/h |
Atomization pressure | 1.5 bar |
Spray rate | 1.1-2.4 g/min |
Table 2: Process parameter for a fluidized bed coater with a Wurster insert. A sustained release drug layer is coated onto placebo Cellets® 100 starter beads.
Drug coating
For drug coating, Cellets® 90 were layered with a suspension of metoprolol succinate in a composition as shown in Table 3.
Material | Concentration (w/w) |
Metoprolol succinate | 22.8 % |
Hypromellose | 0.6 % |
talc (Pharma M) | 4.0 % |
Deionized water | 72.6 % |
Table 3: Composition of metoprolol succinate suspension for drug layering onto Cellets® 90.
The metoprolol succinate-loaded Cellets® 90 microparticles were successfully coated with the Eudragit® NM 30 D based aqueous dispersion, achieving a high product yield of 99 % and a final particle size of less than 200 µm (D50 value).
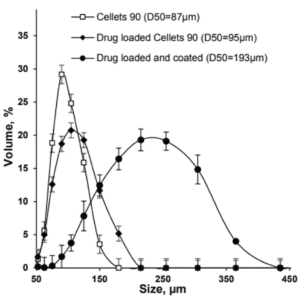
Figure 1: Size distribution of Cellets® 90 as uncoated (empty squares), drug loaded (filled diamonds) and drug loaded and coated (filled circles) particles.
The API loaded and coated starter beads are of high sphericity and show a homogeneous and narrow size distribution, which is shown as a SEM (scanning electron microscope) image in Figure 2.
In dissolution tests, an extended release time of up to 20 hours is obtained and can still be varied by the composition of excipients (Figure 3).
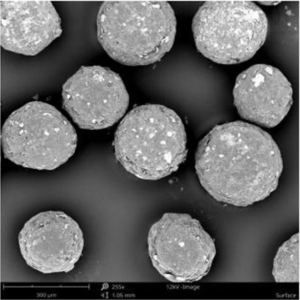
Figure 2: SEM image of drug loaded and coated starter beads. Microparticles show a high level of homogeneity in size distribution.
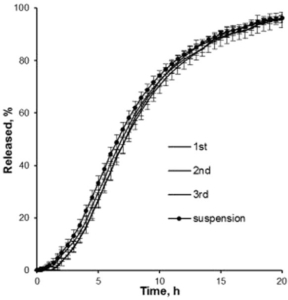
Figure 3: Drug release profiles of three batches of metoprolol succinate loaded and coated Cellets. An extended release of 20 hours is obtained.
Summary
This case study is a short abstract of the publication on microparticle coating by Mohylyuk et al. [1], highlighting the proof of concept for reproducible microencapsulation of a highly water-soluble drug by applying a small quantity of dry powder glidant periodically during Wurster fluidized bed coating. The challenge of particle cohesion in the “down flow” zone was eliminated and a high product yields up to 99% was achieved.
Coated microparticles are in size of less than 200 μm and show a 20 hours sustained drug release profile. These conditions allow the usage in liquid suspensions. Furthermore, the applied technology is scalable. In conclusion, this displays a sustained-release dosage solution, which is suitable for paediatrics and geriatrics with swallowing difficulties.
Acknowledgement
Dr. Fang Liu and her team are gratefully acknowledged for serving content and data for this note.
Fluid Pharma Ltd
Contact: Dr. Fang LIU
College Lane, Hatfield, AL10 9AB, UK
Tel: +44 1707 28 4273
+44 796 3230 628